
Happy SHARK WEEK! Today were diving down, down, down into the deep sea. We’re all familiar with some of the bizarre features of deep-sea fishes, with their long pointy teeth, bioluminescent lures, huge eyes, and flabby bodies. What we almost never see is a deep-sea fish with a skeleton of soft, flexible cartilage instead of bones, or sandpaper-like skin instead of scales.
Despite over 150 years of cumulative scientific sampling and study of the deep sea habitat, sharks that have truly colonized the abyss and beyond have not been discovered.
![The ocean is divided into five major pelagic zones. Nearly all photosynthesis takes place in the epipelagic zone, and light steadily declines to total darkness by the end of the mesopelagic zone (~1000 m). The lower three zones – the bathypelagic, abyssopelagic, and hadopelagic – have relatively similar environments, and are always considered part of the deep sea. Sometimes the midwater (mesopelagic) zone is also put under the umbrella of the deep sea, due the negligible light levels. [Wikimedia Commons]](http://oceanbites.org/wp-content/uploads/2016/06/4.png)
A few chondrichthyans (cartilaginous fish, including sharks), like the frilled shark, cookiecutter shark, bluntnose six gill shark, and the Portuguese dogfish, are reliably established in the 200 m to 3000 m depth range. These sharks are occasionally caught in trawls below 3000 m, but these individuals are generally concluded as outliers, or in the middle of particularly deep dives.
Though respectable (the pressure is up to 5800 psi – twice that of a compressed air SCUBA tank), the depth range of these sharks is a far cry from the distribution of bony fishes (actinopterygians), which have colonized the abyssopelagic (4000 – 6000 m), and even hadopelagic (>6000 m, ocean trenches) zones.
While the total number and diversity of both actinopterygian and chondrichthyan species decline with depth (e.g. there are fewer fish overall and fewer fish species at 1000 m than at 100 m), the trend is much more pronounced in chondrichthyans. If sharks and their relatives had the same species extinction rate with depth as bony fish, their maximum depth would be 7500 m! This means there is thousands of metres of seawater that have fish, but not sharks, and no one’s quite sure why that is the case.
![A frilled shark, the same species used in the header image. This is the first footage of the species in its natural habitat on the Blake Plateau of Georgia, USA. [Wikimedia Commons]](http://oceanbites.org/wp-content/uploads/2016/06/Chlamydoselachus_anguineus_NOOA-300x228.jpg)
So we have ourselves an interesting biogeographical puzzle: why are there so few deep-sea sharks?
The challenges of the deep sea

To call the ocean an alien landscape would be an understatement – we know more about outer space than we do about the deep sea. Dark and vast (88% of the world’s ocean is located >1000 m below the surface), it can be difficult to find a suitable mate (click here to see how angerfishes have solved this problem). It’s also hard to find food, because the deep sea is so nutrient poor, being sustained by the leftovers that fall from the upper zones). Many deep-sea fish have huge, sensitive eyes to help them see in the darkness. Many also have some bioluminescent ability, and these “biological flashlights” can be used not only for communication, but as lures or as camouflage.
The deep sea is cold, typically 1 to 4 degrees Celsius (that’s 34 to 40 Fahrenheit) beyond the midwater (mesopelagic) zone. These extremely low temperatures are challenging to fish and other cold-blooded animals, since the speed of their metabolism is dependent upon the external temperature. The weight of the ocean pushes down upon the deep sea environment, increasing the hydrostatic pressure by 14.5 psi (standard atmospheric pressure at sea level) for every 10 m of depth. In the deepest parts of the ocean, the pressure can have the same crushing force as elephant balancing on a postage stamp!
Class Chondrichthyes: sharks, rays, & chimeras
![Bluntnose six gill shark, at a depth of 1828 ft (557 m), and a temperature of 7.5 degrees Celsius. These fish can reach 5 m (16 ft) in length and is one of the most widely studied deep sea sharks. [Wikimedia Commons]](http://oceanbites.org/wp-content/uploads/2016/06/3.jpg)
Chondrichthyans have some interesting features that distinguish them from bony fishes. Famously, shark skeletons are made of flexible cartilage (like the tip of your nose and your ears) instead of bone. They lack a swim bladder, and instead rely on muscle-powered swimming and stored fat for buoyancy. Unlike fish, sharks and rays match the concentration of dissolved particles in their body fluids to seawater, and retain a waste product (urea) to maintain this osmotic balance. The dermal denticles covering the body of sharks and rays feel like sandpaper, and are anatomically more similar to mammalian teeth than fish scales.
![The dermal denticles of a lemon shark, seen here under a scanning electron microscope, are structurally homologus to vertebrate teeth. [Wikimedia Commons]](http://oceanbites.org/wp-content/uploads/2016/06/6.jpg)
Sharks and their relatives may be excluded from the abyss due to some unique features of their physiology, and how that physiology interacts with the deep sea ecosystem. Here are some of the best theories we have right now to explain why deep-sea chondrichthyans are so rare:
Fat-based buoyancy
Aquatic animals need to offset their body weight to prevent themselves from sinking. Ideally, fish want to be neutrally buoyant (neither sinking nor floating in water) at the depth they choose. One of the ways to do this is with a swim bladder, a gas-filled sac used like a ballast to regulate buoyancy. By inflating or deflating the swim bladder, fish adjust their body density, and this allows them swim at different depths. For example, inflating a swim bladder with gas reduces the overall density of the fish, causing them to float in the water column.
A quirk of sharks and their relatives is that they lack a swim bladder. Instead of accumulating gas for hydrodynamic lift, chondrichthyans pack huge amounts of low-density fats in their liver. There are two major drawbacks to this: not only do sharks need to eat a lot to produce all that fat (40-60% more than a similar-sized bony fish growing at the same rate), but having to retain fat for buoyancy means there is less available to metabolize for energy when food is scarce. While fatty livers work out fine in productive ecosystems like reefs, it may make growth and survival just difficult enough in ocean’s nutrient-poor depths that chondrichthyans are outcompeted by bony fishes.
Urea-based osmoregulation
Multicellular organisms have to carefully control the amount of water inside each cell to prevent shrinking or bursting. This is done by regulating the osmolarity (the concentration of solutes) of the fluid both inside and outside the cell, which controls the net movement of water between these spaces (water always moves from areas of low solute content to areas of high solute content).
![Multicellular organisms need to regulate the volume of their cells to avoid shrinking (which would cause proteins to crowd and interfere with each other) and bursting. This is done by controlling the osmotic pressure in the cells. [Wikimedia Commons]](http://oceanbites.org/wp-content/uploads/2016/06/8.png)
Unlike bony fish, sharks are osmoconformers, meaning their body fluids have a similar concentration of solutes as seawater. But instead of sodium, chloride, and sulfate, the major osmolytes in seawater, sharks use urea and TMAO (trimethylamine N-oxide) to control cell volume. This strategy may limit their absolute maximum depth because of the added stress increasing depth places on the urea/TMAO system.
![TMAO (left) and urea (right) form the basis of shark cell volume regulation. [Wikimedia Commons]](http://oceanbites.org/wp-content/uploads/2016/06/11-300x82.gif)
Urea is relatively non-toxic and energetically cheap, so it’s a good candidate for an osmolyte. However, high concentrations of urea can destabilize proteins. Sharks counter this by also accumulating TMAO in their tissues, an organic compound that can counteract the destabilizing effects of urea. Whether most chondrichthyans produce their own TMAO or get it from eating TMAO-rich food (like molluscs and crustaceans) is unknown.
In shallow-water fishes, about one TMAO molecule is present for every two urea molecules, representing the most thermodynamically favourable ratio (2 urea: 1 TMAO) of these osmolytes. With increasing depth, urea levels decline and TMAO levels increase, and the ratio can be as low as 0.65 urea: 1 TMAO. This is likely due to TMAO pulling “double duty” at depth – counteracting the protein destabilizing effect of both high urea levels and increased hydrostatic pressure. It’s been hypothesized that tissue urea levels can only decrease so much, and/or that TMAO content can only go so high, leading to a maximum depth (pressure) range for animals that rely on this system of osmoregulation.
![Chimaeras or “ghost sharks” diverged from sharks over 400 million years ago. They are found as deep as 2,600 m (8,500 ft). In comparison, the deepest teleost recorded on video is a snailfish in the Mariana Trench, 26,716 feet (8,143 meters) below the surface. [NOAA Okeanos Explorer Program, INDEX-SATAL 2010 via Flickr]](http://oceanbites.org/wp-content/uploads/2016/06/7.jpg)
Food webs & energetics
As relatively large and active animals near the top of the food chain, sharks need a lot of fuel. This isn’t helped by their fatty liver buoyancy strategy, which costs 100-1000 times more energy that inflating a swim bladder. It also doesn’t help that the urea/TMAO osmoregulatory system makes chondrichthyans unusually nitrogen-dependent, meaning they also need a consistent source of nitrogen to maintain osmoregulatory balance.
Being nutrient-hungry in a nutrient-poor ecosystem like the deep sea puts sharks at a significant disadvantage compared to bony fishes. Without energy, everything from foraging to reproduction becomes difficult, if not impossible. It could be that while sharks technically have the capacity to live at depth, they get outcompeted by other animals in practice.
![The Portuguese dogfish is the current record holder for deepest living shark, and has been caught as deep as 3670 m. [Wikimedia Commons]](http://oceanbites.org/wp-content/uploads/2016/06/2.jpg)
Why is the ocean 70% shark free?
The deep sea seems inaccessible to chondrichthyans. Given that 150+ years of cumulative scientific study has yet to find a true abyssal shark, ray, or chimera, it’s unlikely that we’ll ever find one. Life almost always finds a way.
Why this is the case is still being actively discussed, though none of the major hypotheses put forward so far are mutually exclusive. Instead, it seems like our poor chondrichthyans are barred from the deep sea due to interactive physiological and ecological factors that put them at a considerable disadvantage compared to bony fishes.
The deep sea has been put forward as a potential reserve of biomass and species diversity, protected from commercial exploitation due to the technical and financial challenges involved in catching and marketing its inhabitants. With the vast majority of this habitat being closed off to them, sharks and their relatives are particularly vulnerable, especially since their distribution is limited even in friendlier, shallower waters compared to fish.
Further reading
Laxson, C.J., N.E. Condon, J.C. Drazen, and P.H. Yancey. (2011). Decreasing urea: trimethylamine N-oxide ratios with depth in chondrichthyes: a physiological depth limit? Physiological and Biochemical Zoology. 84(5): 494-505. doi: 10.1086/661774
Musick, J.A., and C.F. Cotton. (2014). Bathymetric limits of chondrichthyans in the deep sea: a re-evaluation. Deep Sea Research II. 115: 73-80. doi:10.1016/j.dsr2.2014.10.010. Full text available to download here.
Priede, I.G., R. Frose, D.M. Bailey, O.A. Bergstad, M.A. Collins, J.E. Dyb, C. Henriques, E.G. Jones, and N. King. (2006). The absence of sharks from abyssal regions of the world’s oceans. Proceedings of the Royal Society B. 273: 1435-1441. doi: 10.1098/rspb.2005.3461
Treberg, J.R., and B. Speers-Roesch. (2016). Does the physiology of chondrichthyan fishes constrain their distribution in the deep sea? Journal of Experiment Biology. 219: 615-625. doi: 10.1242/jeb.128108. Full text available to download here.
(Header image of C. anguineus from Wikimedia Commons)
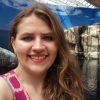
Brittney is a PhD candidate at McMaster University in Hamilton, ON, Canada, and joined Oceanbites in September 2015. Her research focuses on the physiological mechanisms and evolution of the respiratory and metabolic responses of Fundulus killifish to intermittent (diurnal) patterns of hypoxia.