By Marlo Garnsworthy
Originally posted on our sister site, envirobites.org
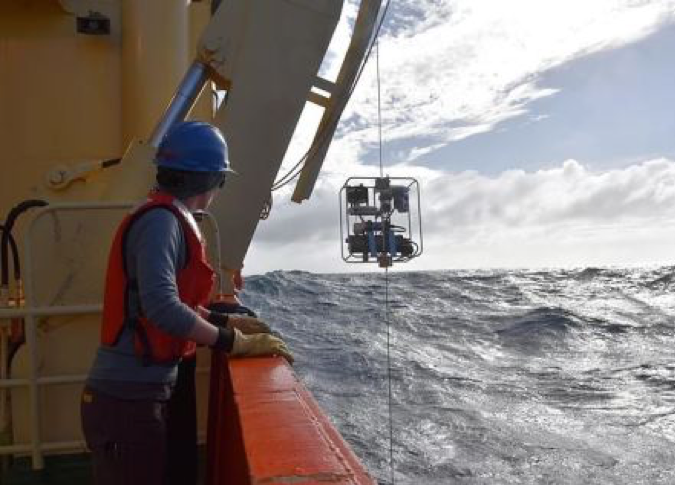
A Wild Ocean Voyage
Waiting on the aft deck for pumps to rise from the sea, the science team is in high spirits. Albatrosses soar on currents in the ship’s wake, the curve of their beaks an enigmatic smile as they glide by. Sunshine glitters on the generous swell, and we’re wearing T-shirts beneath our flotation vests, our iconic United States Antarctic Program parkas hung up for good. It’s our sixth week aboard the RVIB Nathaniel B. Palmer upon the wild Southern Ocean.
Led by oceanographer Dr. Rebecca Robinson (University of Rhode Island, Graduate School of Oceanography) and Dr. Mark Brzezinski (UCSB, Marine Science Institute), our SNOWBIRDS Transect team has been studying how the influence of nitrogen and silicon on the productivity of diatoms, a kind of microalgae, is recorded in sediments.
“We concentrate our efforts on the Southern Ocean, around Antarctica,” says Robinson, “because this region is in direct contact with the deep ocean, where carbon can be stored away from the atmosphere for hundreds to thousands of years.”
The concentration of carbon dioxide in our atmosphere is increasing, unlocked by the burning of fossil fuels. Ice cores have shown that CO2 levels have varied significantly in the past, too. Robinson and her team seek to better understand what controls CO2’s natural variability by developing a “proxy”—a stand in for direct measurements, which enables scientists to reconstruct the climate over longer periods of Earth’s history.
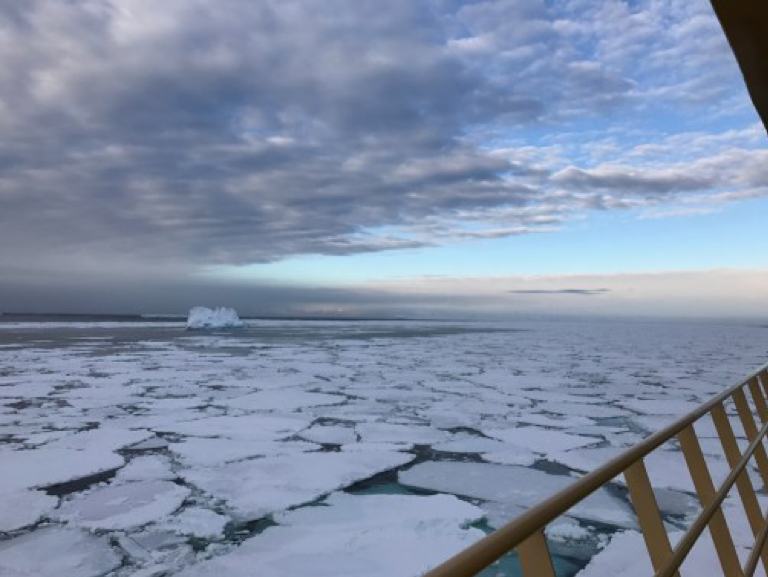
Frigid, Wild, Yet Productive
Our polar oceans play a major role in regulating atmospheric CO2. One might assume the rough, wind-driven, sometimes frozen seas surrounding Antarctica would be averse to life. But for diatoms, conditions are prime. Here, deep, nutrient-rich waters rise to the sea surface and are transported northward. The delivery of nutrients into the upper sunlit zones allows diatoms to thrive.
During the lengthy days of the polar summer, diatoms flourish and “bloom.” They will eventually die and sink to the seafloor, potentially becoming part of a fossil record that scientists “read” to understand the history of diatom productivity in this critical region.
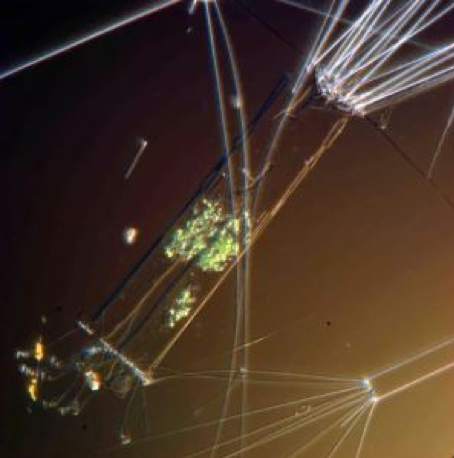
Diatoms and CO2
During photosynthesis, diatoms take up CO2 and other nutrients for growth, and they produce oxygen. Their impact on the global ecosystem is considerable—they’re responsible for about one fifth of the oxygen we breathe. Like other phytoplankton, they form the base of the marine food chain.
Vital Nutrients
The diatom is unique among phytoplankton because its skeleton—called a “frustule”—is made of glass (silica). As diatoms take up nutrients, their intricate structures record the chemistry of the water in which they are growing.
Nitrogen and silicon are essential nutrients for diatoms, and each has multiple isotopes. By sampling live, dead, and fossil diatoms and exploring which isotopes are present and in what concentrations, scientists can determine what conditions were like when the diatoms were growing.
When diatoms die, they take their chemical history with them, sinking slowly through the water column as one component of “marine snow”—which includes bits of dead organisms, dust, and fecal matter, which cluster in aggregations. Many of these sinking diatoms are eaten by other organisms, but some are finally incorporated into the thick, oozy mud that coats the seafloor. This “diatomaceous mud” provides a record of CO2 changes on our planet over many millennia.
But in order to study this mud, we must first retrieve it from the bottom of the world’s roughest ocean.
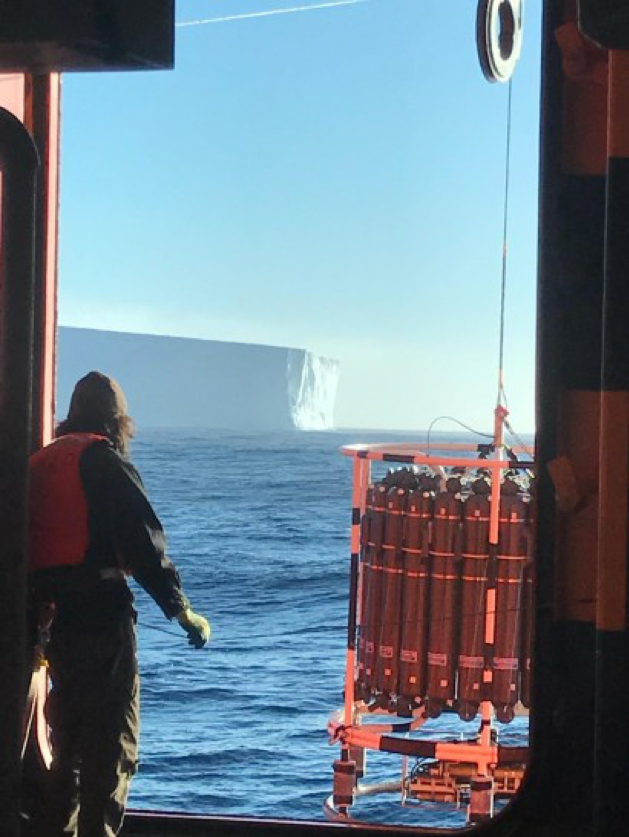
Northward Along 170˚W
We stop at thirteen sites along 170˚W, deploying a sensor called a CTD deep into the water column. It measures salinity, temperature, dissolved oxygen, and fluorescence (an indicator of the amount of chlorophyll in the water).
Above the CTD sits a rosette of bottles, which technicians can “fire” at different depths, causing them to trap water samples, which we analyze for nitrate, silicic acid, and fluorescence.
Technician Pat Kelly uses the CTD’s fluorescence data to determine the chlorophyll maximum (the area of greatest phytoplankton density). He lowers McLane pumps to filter large volumes of seawater for marine snow and packages the filters for analysis back at URI.
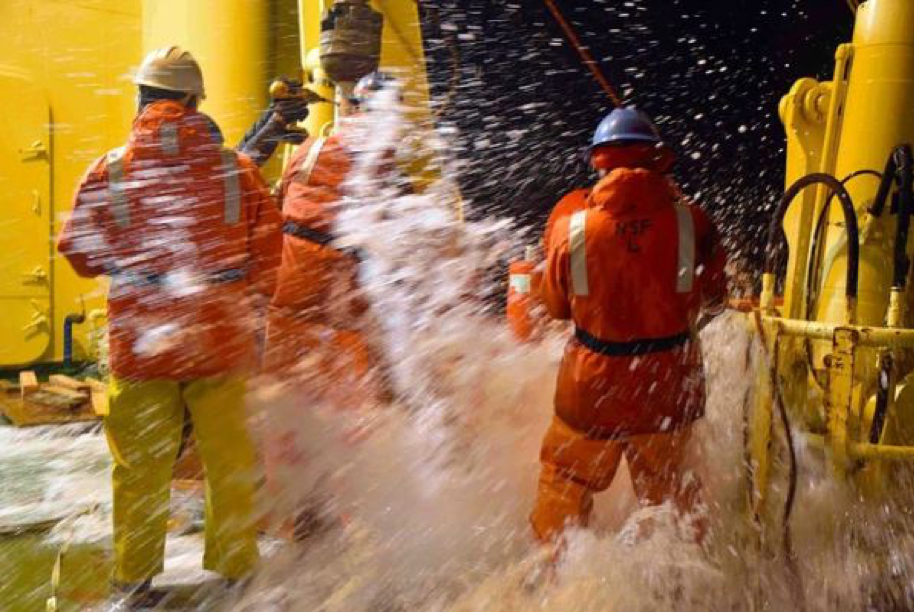
Science Aboard a Heaving Surface
The first rule of living and working aboard a ship in the world’s wildest ocean is: unless you won’t mind it flying across the lab, tie it down. In the lab where Robinson and Kelly are working, as everywhere else aboard, all equipment is secured.
Wave height and general sea state are the main factors affecting science operations. We keep to a tight schedule, but Robinson is ever in close communication with the bridge, who keep the ship steady and onsite. If conditions become too rough, operations cease for the safety of both people and equipment. But even still, operations don’t always run smoothly in the Southern Ocean.
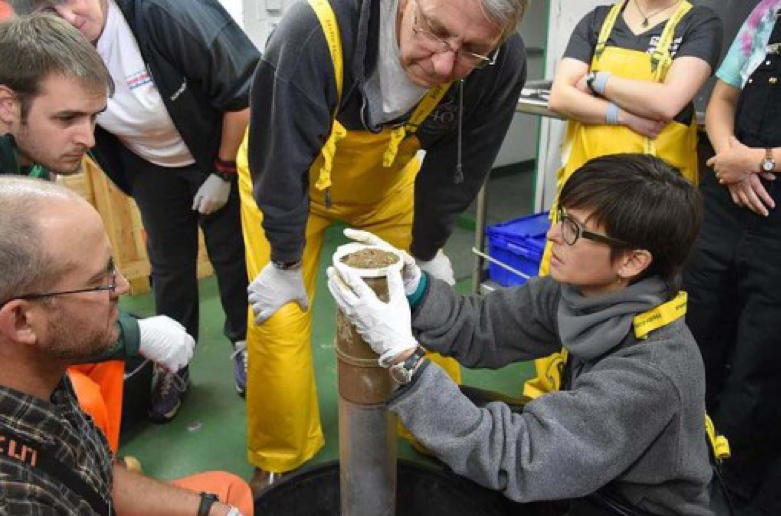
The Trials of Coring
The first time we deploy the megacorer, its tubes return almost full of the pale oozy diatomaceous mud Robinson is seeking. We spend a happy afternoon sectioning, packaging, and labeling the sediment for transport back to the US.
The megacorer’s roundtrip can take several hours, depending on ocean depth and sea state. Each time it approaches the bottom, we eagerly watch the tension on the cable, which expresses as a jagged red line on a monitor. When the megacorer hits the seafloor, tension suddenly decreases, and the corer—hopefully—sinks into and captures mud.
But as we travel into rougher seas and over the region where very dense diatomaceous sediment dominates, the megacorer often returns with little or no mud.
And one night, the cable returns without the megacorer.
As Robinson tells me, “Sending equipment to the bottom of the Southern Ocean and always expecting to get it back requires a certain amount of hubris.” Science in the Southern Ocean also requires adaptability and patience, stamina and tenacity.
Luckily, we have other heavier coring equipment, and we’re ultimately successful in retrieving most of the samples we came for.
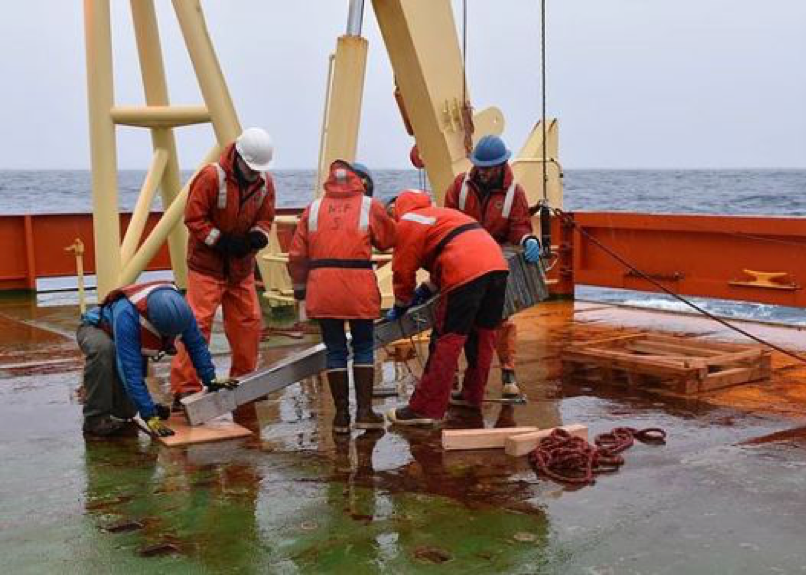
Back in the Lab
Some months later, the water samples, particles, and mud are back in the URI (and UCSB) lab and undergoing analysis. Robinson is particularly interested in ocean circulation and the role of ocean biology in regulating the concentration of atmospheric carbon dioxide.
“Southern Ocean biology has the capacity to take up more CO2, via the biological pump,” says Robinson. “At present, biology does not consume all the major nutrients supplied to the surface ocean. If it did, more CO2 would be drawn out of the atmosphere and potentially delivered to the deep sea.”
Robinson believes her research may help explain natural variations in CO2 concentration and define the most important biological processes in the carbon cycle. “This can help us to think about where anthropogenic CO2might go and the timescales of response.”
Better understanding the carbon cycle may help us prepare for the myriad challenges presented by increasing atmospheric carbon dioxide in the Anthropocene.
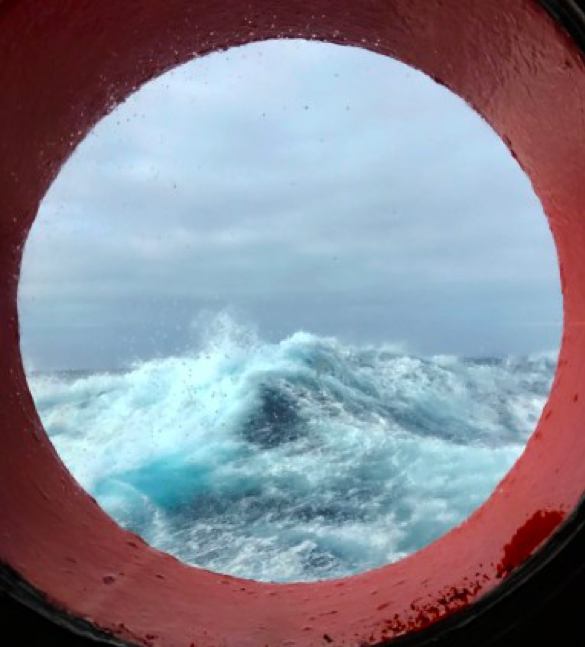
Check out more by the author at:
I am a third year PhD student at the University of Rhode Island Graduate School of Oceanography in the Lohmann Lab. My current research interests include environmental chemistry, water quality, as well as coastal and seabird ecology. When not in the lab, I enjoy diving, surfing, and hanging out with my dog Gypsy.